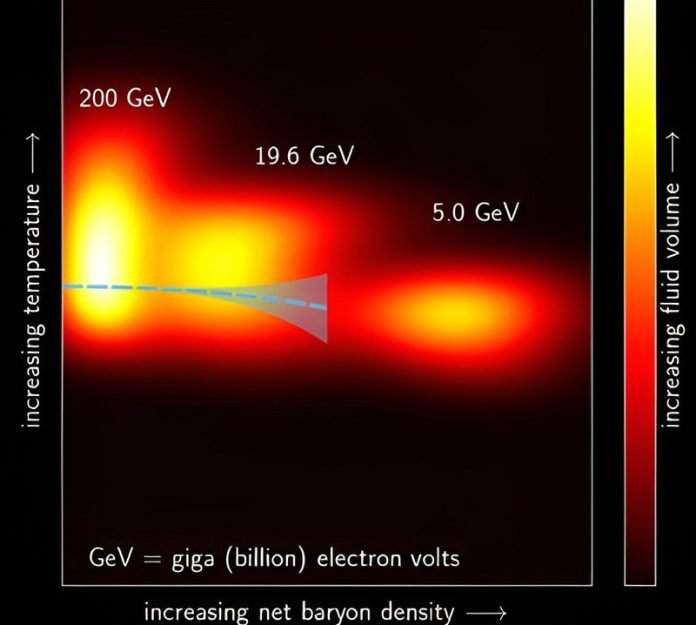
When scientists collide heavy atomic nuclei together, they create a hot, fluid-like soup made of the building blocks of visible matter, called quarks and gluons.
This soup, known as quark-gluon plasma, has very low viscosity, meaning it flows easily.
But how does this “stickiness,” or resistance to flow, change under different conditions?
Researchers have now conducted the first detailed study to understand how the viscosity of this plasma changes across a wide range of collision energies.
Their findings have been published in the journal Physical Review Letters.
The team of theorists considered how the conditions change as the nuclei pass through each other during the collision.
They found that the viscosity of the plasma increases with the net-baryon density—the number of baryons (particles like protons and neutrons made of three quarks) compared to antibaryons (particles with opposite properties created in the collision).
Using data from experiments where gold nuclei were collided at different energies, the researchers adjusted their simulations to fit the experimental results.
Their calculations showed that as the net-baryon density increases, so does the viscosity of the plasma. This finding matches some, but not all, previous theoretical predictions.
Looking ahead, scientists will use this theoretical framework to incorporate more data from various collision energies.
These expanded simulations will not only help to understand viscosities but will also map out the entire phase diagram of nuclear matter. This diagram shows how nuclear matter changes from a solid, liquid, gas, or plasma depending on temperature and baryon density.
This research utilized cutting-edge simulations that model the viscous fluid dynamics in all three spatial dimensions, along with newly developed models for the initial stages of the collisions. These simulations describe heavy ion collisions at the Relativistic Heavy Ion Collider (RHIC), a facility operated by the Department of Energy, over a wide range of collision energies.
By including the evolution of the initial state, the researchers could continuously generate fluid nuclear matter as the nuclei pass through each other. This approach is particularly important for lower energy collisions, where assuming an instantaneous collision isn’t accurate.
The study was conducted by a team from Brookhaven National Laboratory, Lawrence Berkeley National Laboratory, the University of California Berkeley, and Wayne State University.
They used this versatile model to perform detailed event-by-event calculations, considering the fluctuations in the initial geometry of the colliding nuclei and the resulting shape of the produced fireball.
By varying and constraining the model parameters, which include the viscosities of the produced matter and properties of the initial state, the researchers performed a statistical analysis using data from RHIC’s Beam Energy Scan (BES).
This analysis, based on 5 million simulated collision events, can now be compared to pure theoretical calculations and applied to future experiments at RHIC and the Facility for Antiproton and Ion Research (FAIR) in Europe.