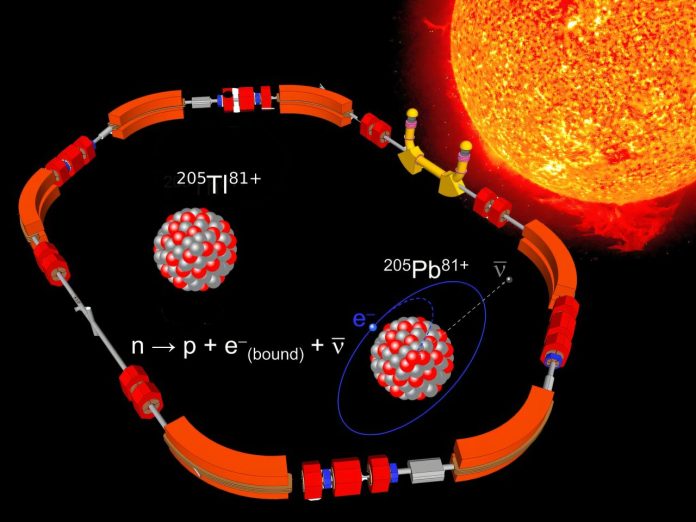
The sun, the powerhouse of life on Earth, generates its energy through nuclear fusion and constantly releases neutrinos—tiny particles that act as messengers of its inner workings.
While modern detectors can track the sun’s activity today, many mysteries remain about its stability over millions of years.
These questions are critical for understanding changes in Earth’s climate and the evolution of life.
To uncover these long-term solar patterns, scientists are working on the LORandite EXperiment (LOREX), an ambitious project that aims to study the sun’s behavior over the past four million years.
Central to this effort is the mineral lorandite, which contains thallium (Tl). When neutrinos from the sun hit thallium atoms in lorandite, they transform into lead (Pb) atoms.
The isotope 205Pb, with a half-life of 17 million years, acts as a time capsule, storing a record of solar neutrino interactions across millennia.
To advance the LOREX project, researchers needed precise data about how neutrinos interact with thallium-205 (205Tl).
However, measuring this directly is impossible with current technology. Instead, an international team devised a creative solution at the GSI/FAIR laboratory in Darmstadt, Germany.
Using the unique Experimental Storage Ring (ESR) at GSI/FAIR, scientists studied a related process: the beta decay of fully ionized 205Tl81+ ions into 205Pb81+.
By observing this decay, they could calculate the nuclear matrix element, a critical value for determining the neutrino cross-section (how often neutrinos interact with thallium).
The experiment required cutting-edge accelerator technology to produce pure beams of 205Tl81+ ions. These ions were created through nuclear reactions in GSI/FAIR’s Fragment Separator (FRS) and stored in the ESR long enough to measure their decay.
“Thanks to decades of technological advancements, we were able to produce an intense and pure beam of 205Tl81+ ions and measure their decay with high precision,” said Professor Yuri A. Litvinov, spokesperson for the project.
The team found that the half-life of this decay is 291 days (+33/-27), a crucial value for interpreting the neutrino interactions recorded in lorandite minerals.
With this new data, the LOREX project can estimate the concentration of 205Pb in lorandite and provide a clearer picture of the sun’s behavior over millions of years.
This knowledge could also help scientists explore links between solar activity and Earth’s climate changes during the same period.
“This experiment shows how one precise measurement can answer big questions about the sun and its impact on our planet,” said Dr. Ragandeep Singh Sidhu, the lead author of the study.
The findings, published in Physical Review Letters, highlight the power of nuclear astrophysics in uncovering the universe’s secrets. They also demonstrate how innovative methods can address challenges that once seemed impossible to solve.
The researchers dedicated this milestone work to the memory of key contributors whose efforts were instrumental in making this achievement possible. Their legacy lives on in the groundbreaking insights into our sun’s long-term history.