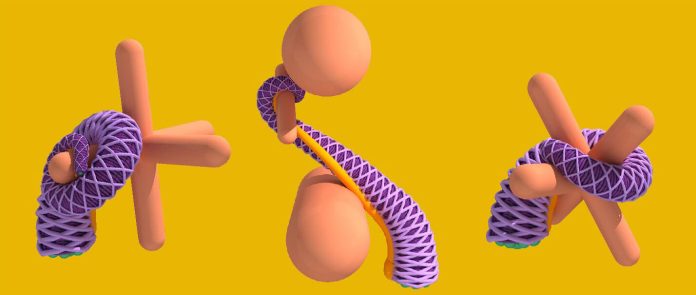
A team of engineers has developed a groundbreaking computer model that captures the complex muscle structure of an octopus arm, offering valuable insights for creating flexible, soft robots inspired by these fascinating creatures.
The research, published in the Proceedings of the National Academy of Sciences, explores the unique movement capabilities of an octopus’s arm and opens doors to a new kind of “cyberoctopus” robot design.
The octopus is known for its unmatched ability to move with extreme flexibility and precision. Unlike most animals, including humans, which rely on a central brain for control, the octopus has distributed “mini-brains” along each arm.
This unique feature allows each arm to act independently while still coordinating with the others. The arm’s motion is extremely versatile, described as having almost infinite degrees of freedom, which makes it incredibly complex to understand and replicate.
The team behind this new model, led by Associate Professor Mattia Gazzola at the University of Illinois Urbana-Champaign, has been working on this project since 2019.
Their aim was to decode the “secret” behind the octopus’s movements to help design advanced robotic systems that could mimic these movements. Gazzola explained, “The general motivation is to find a way to control a complex system with many degrees of freedom while avoiding the need for expensive computations.”
To build the model, the researchers used MRI imaging and biomechanical data to recreate the structure of an octopus arm with nearly 200 intertwined muscle groups.
This detailed model allows for a deeper understanding of how the arm moves, twists, and stretches with great precision.
They also studied the movements of a live octopus by observing it through an experiment. The octopus was placed on one side of a Plexiglas sheet with a hole large enough for only one arm to reach through.
By placing an object on the other side, the team could capture video footage of the octopus reaching and manipulating the object, observing how it naturally moved its arm.
One of the key insights from this work was understanding how the arm’s complex movements could be controlled with relatively simple muscle contractions.
Instead of needing to control each tiny movement, the researchers used mathematical methods involving topology and differential geometry to explain the arm’s shape and motion.
“We created simple muscle activation templates that could achieve complex 3D motion,” Gazzola explained. “We focused on two main topological properties—writhe and twist—which are controlled by specific muscle groups.
When these muscle groups work together, they produce a third property that describes the arm’s 3D changes in shape.”
The computer model that the team developed has significant implications beyond biology. It’s a powerful tool for roboticists who aim to design robots with flexible and dynamic movement. “Our model acts as a useful testbed for roboticists to try out their algorithms,” said Professor Prashant Mehta, who collaborated on the project.
This work reflects a large collaborative effort across multiple institutions. Ph.D. candidate Arman Tekinalp, a key member of the team, shared his excitement about learning from the octopus to inspire the design of soft robots.
Mechanical engineering students from various groups, including those of Assistant Professor Noel Naughton at Virginia Tech, contributed to the study, demonstrating the value of interdisciplinary collaboration.
Looking ahead, the team hopes to expand their model to control all eight arms of the octopus in a coordinated way, similar to how an octopus would use multiple arms to handle different objects at once. They also plan to develop a robotic prototype to test their findings in real-life applications.
While this work has greatly advanced understanding, the researchers acknowledge there is more to learn. “We have a strong theoretical grasp, but we want to create an automated system that allows our octopus model to learn and perform tasks on its own,” Gazzola said.
As Tekinalp graduates and moves on to a postdoctoral position at the University of Maryland, College Park, the project will continue with new team members eager to uncover the mysteries of octopus movement.
This model marks a significant milestone in both biology and robotics, potentially bringing us closer to a future where robots can match the graceful, flexible movements of nature’s most fascinating creatures.