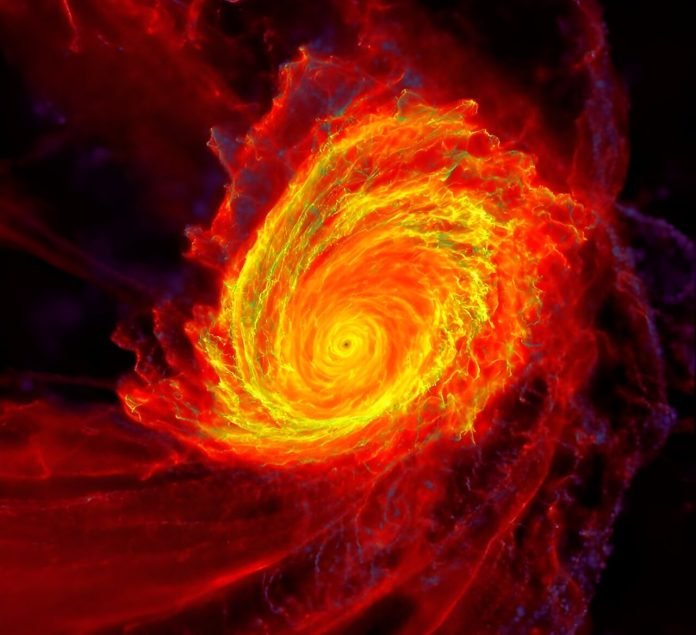
Astrophysicists at Caltech have achieved a groundbreaking feat: they have simulated the journey of primordial gas from the early universe to the moment it forms a disk of material feeding a supermassive black hole.
This new simulation changes long-held ideas about these disks and paves the way for new discoveries about the growth and evolution of black holes and galaxies.
Phil Hopkins, the Ira S. Bowen Professor of Theoretical Astrophysics at Caltech, led the team. He explains that this work is the result of several years of collaboration between two large research groups at Caltech.
The first group, known as FIRE (Feedback in Realistic Environments), studies large-scale questions like how galaxies form and interact.
The second group, called STARFORGE, focuses on smaller scales, such as how stars form within clouds of gas. However, there was a significant gap between these two areas of study.
“For the first time, we have bridged that gap,” Hopkins says.
The researchers built a simulation with a resolution more than 1,000 times greater than the previous best in the field.
To their surprise, the simulation revealed that magnetic fields play a much larger role than previously thought in forming and shaping the massive disks of material that swirl around and feed supermassive black holes.
“Our theories told us the disks should be flat like crepes,” Hopkins says. “But astronomical observations reveal that the disks are actually fluffy—more like an angel cake. Our simulation helped us understand that magnetic fields are propping up the disk material, making it fluffier.”
In the new simulation, researchers performed a “super zoom-in” on a single supermassive black hole. These gigantic objects, which lie at the heart of many galaxies, including our own Milky Way, have masses ranging from thousands to billions of times that of the sun. They exert a massive gravitational pull on anything that comes near them.
Astronomers have known for decades that gas and dust pulled in by these black holes form a rapidly swirling disk called an accretion disk. As the material gets close to falling in, it radiates a huge amount of energy, shining brightly. These active supermassive black holes are called quasars. However, much about these disks remains unknown.
While previous imaging efforts like the Event Horizon Telescope have captured disks around nearby black holes, these disks are more tame than those around quasars. To visualize what happens around more active and distant black holes, astrophysicists use supercomputer simulations.
They input various physical laws—from gravity to the behavior of dark matter and stars—into thousands of computing processors working in parallel.
Creating a simulation that covers all relevant scales, from the large-scale universe to a single accretion disk around a black hole, was a huge challenge. It required a code that could handle all the necessary physics. Hopkins explains that previous codes could handle either the large-scale or the small-scale physics but not both.
The team used a code called GIZMO for both large- and small-scale simulations. They built the FIRE project so that all the physics could work with the STARFORGE project and vice versa. This modular approach allowed them to simulate a black hole about 10 million times the mass of our sun, starting from the early universe. The simulation then zooms in on the black hole as it pulls in a stream of material, forming an accretion disk.
“In our simulation, we see this accretion disk form around the black hole,” Hopkins says. “We would have been excited just to see that, but what was very surprising was that the simulated disk doesn’t look like what we’ve thought for decades it should look like.”
In the 1970s, scientists believed that thermal pressure—the pressure from the heat of the gas—played the dominant role in preventing accretion disks from collapsing under the black hole’s gravity. They thought magnetic fields had a minor role. However, the new simulation found that the pressure from magnetic fields is 10,000 times greater than the pressure from the heat of the gas.
“So, the disks are almost completely controlled by the magnetic fields,” Hopkins says. “The magnetic fields prop up the disks and make the material puffy.”
This discovery changes many predictions about accretion disks, including their mass, density, thickness, and the speed at which material moves into the black hole. It even affects their shape.
Hopkins hopes this new ability to bridge the gap in scales for cosmological simulations will open many new avenues of research. For example, what happens when two galaxies merge? What types of stars form in dense regions of galaxies? What might the first generation of stars in the universe have looked like?
“There’s just so much to do,” he says.
This new simulation marks a significant step forward in our understanding of the universe, showing how powerful the combination of advanced computing and collaborative efforts can be in unlocking the secrets of black holes and their role in the cosmos.