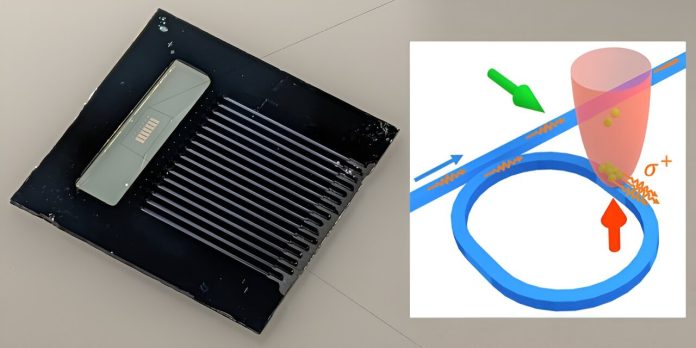
Researchers at Purdue University have achieved a groundbreaking feat by trapping alkali atoms, specifically cesium, on an integrated photonic circuit.
This circuit behaves like a transistor for photons, the smallest units of light, similar to how electronic transistors control the flow of electricity.
This discovery could pave the way for building a quantum network using cold-atom integrated nanophotonic circuits.
The team, led by Chen-Lung Hung, an associate professor of physics and astronomy at Purdue University, published their findings in the journal Physical Review X.
Hung explains, “We developed a technique to use lasers to cool and tightly trap atoms on an integrated nanophotonic circuit.
In this circuit, light travels through a tiny photonic ‘wire,’ or waveguide, that is over 200 times thinner than a human hair.”
These atoms are cooled to an incredibly low temperature, just a tiny fraction above absolute zero, where they are almost completely still.
At this extremely cold temperature, the atoms can be captured by a “tractor beam” and placed very close to the photonic waveguide, at a distance about the size of a virus. At this proximity, the atoms interact efficiently with the photons traveling through the waveguide.
Using advanced nanofabrication tools at the Birck Nanotechnology Center, the team created a circular photonic waveguide called a microring resonator. This structure, about three times smaller than a human hair, allows light to circulate within it and interact with the trapped atoms.
The researchers demonstrated that this atom-coupled microring resonator can act as a “transistor” for photons.
By controlling the state of the trapped atoms, they can gate, or regulate, the flow of light through the circuit. If the atoms are in one state, photons can pass through the circuit; if they are in another state, the photons are blocked. The stronger the interaction between the atoms and the photons, the more efficient this gate becomes.
Xinchao Zhou, a graduate student involved in the research, stated, “We have trapped up to 70 atoms that can collectively interact with photons and control their transmission on an integrated photonic chip. This achievement has never been realized before.”
The research team, all based at Purdue University in West Lafayette, Indiana, includes Tzu-Han Chang, who designed and fabricated the circuit, and Hikaru Tamura, who helped set up the critical parts of the experiment. The study was supervised by Chen-Lung Hung.
“Our technique, detailed in the paper, allows us to efficiently cool many atoms on an integrated photonic circuit,” says Zhou. “Once trapped, these atoms can collectively interact with light traveling through the photonic waveguide, similar to how synchronized rowers can move a boat faster.”
This approach is unique because the atoms are identical and can couple with light in the same way, building phase coherence. This means the atoms can collectively interact with light with greater strength. Solid-state emitters in a photonic circuit, on the other hand, often have slight differences that make it hard for them to build phase coherence.
This research demonstrates a potential for creating a quantum network based on cold-atom integrated nanophotonic circuits. Such a network could manipulate and store quantum information in trapped atoms, known as qubits. These qubits could then transfer quantum information into photons, which could travel through the photonic circuit and communicate with other atom-coupled circuits.
The team has been working on this research for several years and plans to continue exploring new directions.
They aim to arrange trapped atoms in an organized array along the photonic waveguide, allowing for stronger interactions and improved photon storage in a quantum system. They also plan to cool the atoms even closer to absolute zero to study new states of quantum matter and enhance radiative coupling for creating cold molecules.
The future for this research is promising, with many exciting avenues to explore and potential breakthroughs in quantum computing and communication on the horizon.