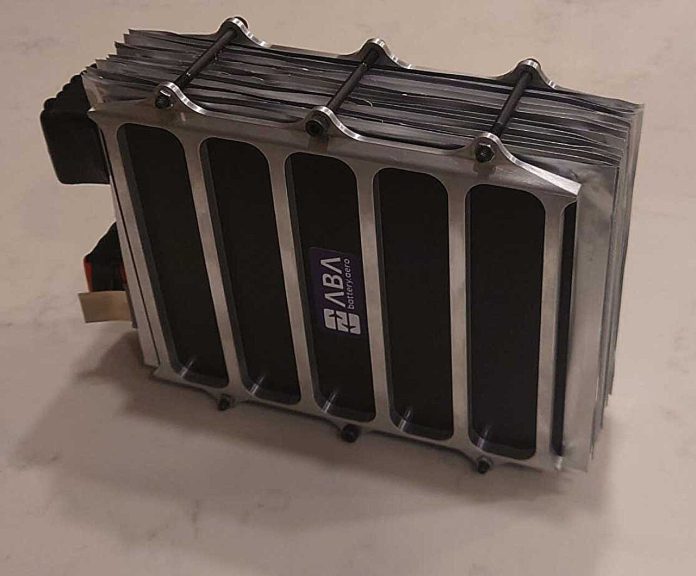
A recent breakthrough in battery technology could significantly improve the power delivery for electric aircraft, especially during critical phases like takeoff and landing.
This innovation, led by researchers from Lawrence Berkeley National Laboratory in collaboration with the University of Michigan, addresses a key challenge faced by electric aircraft: maintaining high power output even when the battery charge is low.
The research team, which included expertise from U-M’s aerospace engineering professor Venkat Viswanathan, published their findings in the journal Joule.
Viswanathan had previously identified the problem in a 2018 study.
He explained, “Both takeoff and landing require high power, and landing is more challenging because you’re not fully charged. To get high power, you have to reduce all the resistances that affect power delivery.”
While electric vehicle (EV) batteries mainly focus on maintaining their range, the needs of aircraft batteries are different.
“In an electric vehicle, you focus on capacity fade over time,” said Youngmin Ko, the lead author of the study and a postdoctoral researcher at Berkeley Lab’s Molecular Foundry.
“But for aircraft, it’s the power fade that’s critical—the ability to consistently achieve high power for takeoff and landing.”
Both capacity fade and power fade occur when lithium ions struggle to move in and out of the battery electrodes.
Capacity fade is influenced by the quantity of lithium ions, whereas power fade is affected by the speed of ion movement. Over time, corrosion on the electrodes can hinder this movement, reducing the battery’s power output.
Brett Helms, the corresponding author and a senior scientist at Berkeley Lab, led the team in exploring how the battery electrodes and electrolyte interact. They used a method borrowed from biology, often referred to as “omics,” which examines the components within cells. In this case, the team tested different electrolyte chemistries to observe subtle changes during the battery’s charging and discharging cycles.
Traditionally, power fade was thought to be caused by issues on the battery’s negative side, where lithium metal is highly reactive.
However, the team discovered that damaging molecules were forming near the positive side, which in this study was made of nickel-manganese-cobalt oxide. These molecules reacted with the positive electrode, causing it to crack and corrode, thereby reducing lithium movement and power delivery.
Ko noted, “It was a non-obvious outcome. We found that mixing salts in the electrolyte could suppress the reactivity of typically reactive species, which formed a stabilizing, corrosion-resistant coating.”
The company 24M, based in Cambridge, MA, built a test cell using this new chemistry and sent it to And Battery Aero in Palo Alto, CA. And Battery Aero, a startup co-founded by Viswanathan and his former Ph.D. student Shashank Sripad, tested the cell in realistic flight conditions.
The new cell maintained the power-to-energy ratio needed for electric flight four times longer than conventional batteries.
“Heavy transport sectors, including aviation, have been underexplored in terms of electrification,” said Helms. “Our work redefines what’s possible, pushing the boundaries of battery technology to enable deeper decarbonization.”
Next, 24M will build a complete battery that And Battery Aero will test on a propeller stand, simulating repeated flight sequences. The team plans to attempt an electric flight test with these batteries next year.
The researchers also aim to expand the use of “omics” in battery research to better understand and tailor battery performance for various transportation and grid applications. Viswanathan and Sripad have a financial interest in And Battery Aero, reflecting their commitment to advancing this technology.