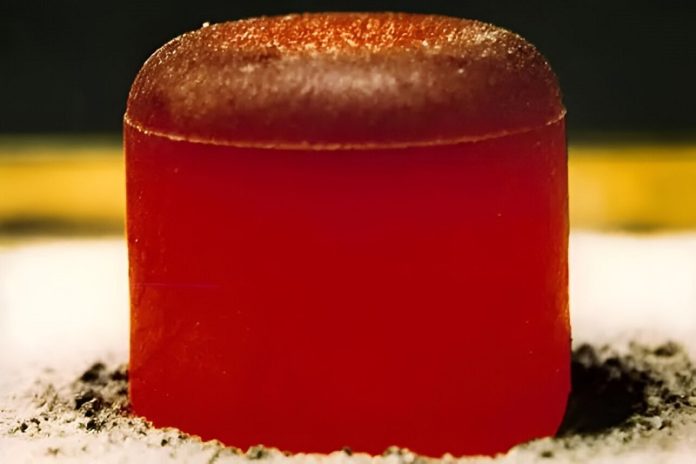
Have you ever wondered what a spacecraft and a pacemaker have in common?
Both are powered by plutonium-238 (238Pu), a special material known for its ability to generate heat.
Recent research has introduced a new method that could make the production of 238Pu more efficient and less costly, potentially transforming space exploration and medical technology.
The findings, published in the journal Nuclear Science and Techniques, describe a high-resolution model that increases the yield of 238Pu by nearly 20% in high-flux reactors.
This breakthrough could significantly lower the costs of producing this important isotope.
A group of nuclear scientists from Shanghai Jiao Tong University and the Nuclear Power Institute of China have developed methods that improve the precision of 238Pu production.
Their techniques—filter burnup, single-energy burnup, and burnup extremum analysis—resulted in an 18.81% increase in yield.
These improvements allow for a detailed resolution of about 1 electron volt (eV), eliminating many of the theoretical approximations previously used in the field.
“Our work not only advances isotopic production technologies but also offers a new way to approach nuclear transmutation in high-flux reactors,” said Qingquan Pan, the study’s lead researcher.
The importance of Plutonium-238
Plutonium-238 plays a crucial role in powering devices that traditional batteries can’t support, such as spacecraft and medical devices like pacemakers.
However, producing 238Pu has been challenging due to inefficiencies and high costs, largely because of a lack of precise models.
The new method analyzes the complex reactions within nuclear reactors, creating a model that not only improves production but also reduces the impact of gamma radiation. This makes the process safer and more environmentally friendly.
How It Works
The production of 238Pu involves irradiating Neptunium-237 (Np-237) in a reactor. This method has the advantage of producing low radioactive contamination. During irradiation, various new nuclides are produced, and multiple nuclear reactions occur, forming a complex transformation process.
The researchers compared three methods: filter burnup, single-energy burnup, and burnup extremum analysis. The first two methods provide insights into how the energy spectrum affects nuclear reactions, while the third method looks at how changes over time influence production efficiency. Together, these techniques enable precise control and optimization of neutron reactions within reactors.
This research has vast implications. Improved 238Pu production can directly support devices operating in harsh environments. “This model could significantly impact future space missions by providing longer-lasting power for spacecraft and enhancing the reliability of medical devices like pacemakers,” said Pan.
The new production process means more 238Pu can be produced with fewer resources, reducing environmental impact and improving the safety of production facilities.
Looking ahead, the research team plans to expand their model’s applications. “Our next steps involve refining target design, optimizing the neutron spectrum used in production, and constructing dedicated irradiation channels in high-flux reactors,” Pan added.
These developments could streamline the production of 238Pu and be adapted for other scarce isotopes, promising widespread benefits across various scientific and medical fields.
The creation of a high-resolution neutronics model marks significant progress in nuclear science. This model’s application to other scarce isotopes could lead to major advancements in energy, medicine, and space technology.
As the world moves towards more sophisticated energy solutions, Pan and his team’s work highlights the crucial role of innovative nuclear research in building a sustainable and technologically advanced future.