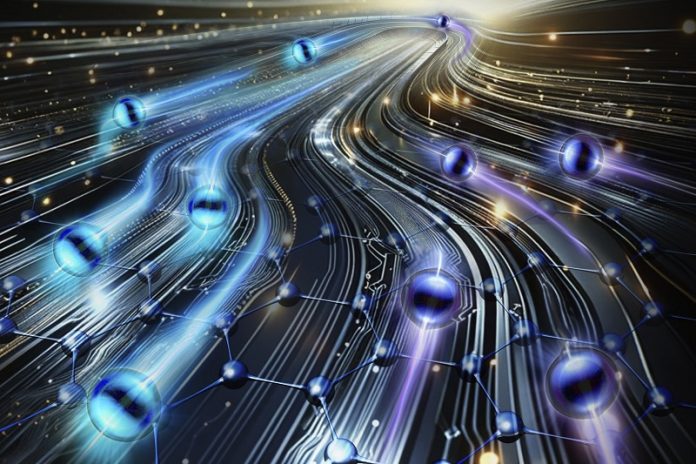
MIT physicists and their colleagues have created a remarkable five-lane superhighway for electrons, potentially paving the way for ultra-efficient electronics.
Their discovery, detailed in the May 9 issue of Science, is part of a series of significant findings involving a unique form of pencil lead material.
“This discovery has direct implications for low-power electronic devices because no energy is lost during the propagation of electrons, which is not the case in regular materials where the electrons are scattered,” said Long Ju, an assistant professor in the MIT Department of Physics and the corresponding author of the paper.
Imagine cars traveling smoothly down an open highway compared to those navigating through busy neighborhoods.
On the highway, cars move freely without interruptions, while in neighborhoods, they can be slowed or stopped by other drivers. Similarly, this new material allows electrons to move without being scattered, ensuring no energy loss.
The groundbreaking material, called rhombohedral pentalayer graphene, was discovered two years ago by Ju and his team. “We found a goldmine, and every scoop is revealing something new,” said Ju, who is also affiliated with MIT’s Materials Research Laboratory.
In a previous paper published in Nature Nanotechnology last October, the team reported three significant properties of rhombohedral graphene.
For instance, they showed that it could allow the smooth movement of electrons around the edges of the material but not through the middle, creating a superhighway. However, this required a strong magnetic field, much stronger than Earth’s.
In their latest work, the team managed to create the superhighway without any magnetic field.
Graphite, commonly known as pencil lead, consists of layers of graphene—sheets of carbon atoms arranged in a hexagonal pattern, resembling a honeycomb. Rhombohedral graphene is made of five layers of graphene stacked in a specific overlapping order.
Ju and his colleagues isolated rhombohedral graphene using a unique microscope that Ju built at MIT in 2021. This microscope can quickly and inexpensively determine various important characteristics of a material at the nanoscale. The pentalayer rhombohedral graphene they studied is only a few billionths of a meter thick.
For their latest discovery, the team added a layer of tungsten disulfide (WS2) to the original system. “The interaction between the WS2 and the pentalayer rhombohedral graphene resulted in this five-lane superhighway that operates at zero magnetic field,” explained Ju.
The phenomenon discovered in rhombohedral graphene, allowing electrons to travel without resistance at zero magnetic field, is known as the quantum anomalous Hall effect. This differs from superconductivity, a phenomenon where electrons also travel without resistance but in entirely different materials.
While superconductors were discovered in the 1910s, it took nearly a century of research to make them work at temperatures suitable for practical applications. The current record is still well below room temperature.
Similarly, the rhombohedral graphene superhighway operates at about 2 Kelvin, or -456 Fahrenheit. “It will take a lot of effort to elevate the temperature, but as physicists, our job is to provide the insight; a different way for realizing this [phenomenon],” said Ju.
The discoveries involving rhombohedral graphene resulted from meticulous research that wasn’t guaranteed to succeed. “We tried many recipes over many months,” said graduate student Tonghang Han, “so it was very exciting when we cooled the system to a very low temperature and [a five-lane superhighway operating at zero magnetic field] just popped out.”
Ju added, “It’s very exciting to be the first to discover a phenomenon in a new system, especially in a material that we uncovered.”
This breakthrough could lead to significant advancements in the development of low-power electronic devices, making our technology more efficient and sustainable.
Source: MIT.