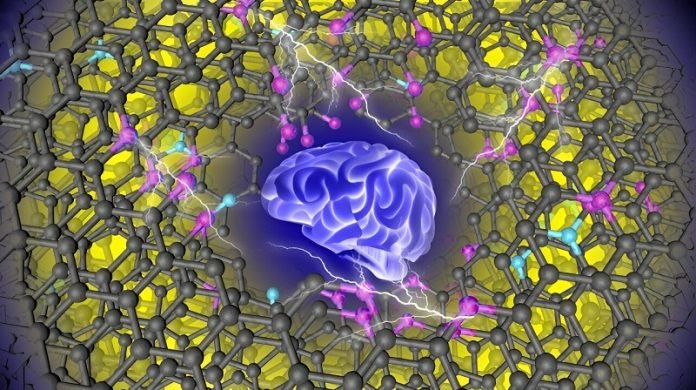
In a remarkable advancement, chemists at the Department of Energy’s Oak Ridge National Laboratory (ORNL) have developed a carbon-based supercapacitor material that holds four times more energy than the best available commercial products.
This new material could revolutionize energy storage in supercapacitors, enhancing the efficiency of regenerative brakes, power electronics, and auxiliary power supplies.
Tao Wang from ORNL and the University of Tennessee, Knoxville, led this innovative study.
Titled “Machine-learning-assisted material discovery of oxygen-rich highly porous carbon active materials for aqueous supercapacitor,” it was published in Nature Communications and co-authored by chemist Sheng Dai of ORNL and UTK.
This new material sets a record for energy storage in porous carbon. “This is the highest recorded storage capacitance for porous carbon,” said Dai, who, with Wang, conceptualized and conducted the experiments.
The research was conducted at the Fluid Interface Reactions, Structures, and Transport Center (FIRST), an ORNL-led Energy Frontier Research Center.
Here, the team explored fluid-solid interface reactions crucial for capacitive electrical energy storage – the ability of a material to collect and store electrical charge.
While batteries are well-known for converting chemical energy to electrical energy and storing it, capacitors store energy as an electric field, similar to static electricity.
They can’t store as much energy as batteries but recharge quickly and maintain their charge-holding capacity.
Supercapacitors, like those in some electric buses, store more charge than regular capacitors and charge/discharge faster than batteries.
In commercial supercapacitors, two electrodes (an anode and a cathode) are separated and immersed in an electrolyte.
At the interface between the electrolyte and the carbon of the electrodes, double electrical layers reversibly separate charges. Porous carbons, with their large surface areas, are typically used for making these electrodes.
The ORNL-led study employed machine learning, a form of artificial intelligence that learns from data to improve outcomes, to discover this exceptional material.
Researchers from the University of California, Riverside, a FIRST partner, built an artificial neural network model and trained it to develop this “dream material” for energy delivery.
The model predicted the highest capacitance for a carbon electrode would be 570 farads per gram if the carbon were co-doped with oxygen and nitrogen.
Following this, Wang and Dai designed an extremely porous doped carbon providing vast surface areas for electrochemical reactions, and Wang synthesized this novel material.
The carbon was activated to create more pores and add functional chemical groups, using a process developed by Dai using sodium amide as the activation agent.
This method works at a lower temperature (around 600°C) compared to the industry standard (around 800°C), preserving more functional groups.
The result was a material with a capacitance of 611 farads per gram, significantly higher than typical commercial materials. The material’s surface area was also one of the highest recorded for carbonaceous materials, over 4,000 square meters per gram.
This breakthrough was achieved rapidly thanks to the data-driven approach, condensing what would have taken a year or more into just three months.
The key was developing two types of pores – mesopores (2 to 50 nanometers) and micropores (smaller than 2 nanometers). This combination provided both a high surface area for energy storage and efficient channels for electrolyte transport.
Miaofang Chi and Zhennan Huang at the Center for Nanophase Materials Sciences used scanning transmission electron microscopy to characterize the mesopores, though the micropores were too small to see.
The material resembled a golf ball with deep dimples representing mesopores, and micropores existed between these dimples.
Quasielastic neutron scattering experiments at the Spallation Neutron Source, a DOE facility at ORNL, were conducted by Murillo Martins and Eugene Mamontov to track the electrolyte’s movement in these pores. They found that the electrolyte moved quickly in mesopores and slower in micropores.
Wang also quantified the capacitance contributions from different pore sizes and oxidation-reduction reactions at their surfaces. Mesopores doped with oxygen and nitrogen contributed most to the overall capacitance.
Further studies of the material’s properties were conducted by Jinlei Cui and Takeshi Kobayashi at Ames National Laboratory and Bishnu Thapaliya at ORNL and UTK, who used nuclear magnetic resonance and Raman analysis, respectively.
This research opens new avenues in the development and optimization of carbon materials for supercapacitor applications.
With even more data now available for training machine learning models, scientists like Wang are optimistic about pushing the boundaries of carbon supercapacitors even further. This success exemplifies the power of data-driven approaches in advancing technology.
Source: Oak Ridge National Laboratory.