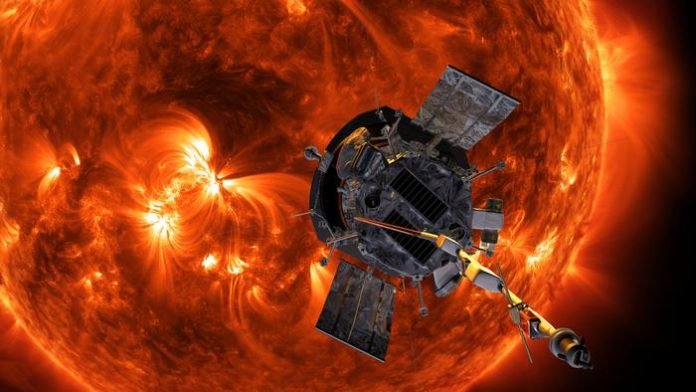
NASA’s Parker Solar Probe (PSP) has embarked on an extraordinary mission to explore the Sun, and its latest findings have unveiled captivating details about the solar wind.
The solar wind, a constant stream of charged particles emitted by the Sun, has long fascinated scientists.
By flying closer to the Sun’s surface than any previous spacecraft, the Parker Solar Probe has now observed the fine structure of the solar wind, providing crucial insights into its origins.
Imagine standing in front of a showerhead, feeling the forceful blast of water on your face. Now picture this scenario in space: the solar wind, akin to the water from the showerhead, emerges from the Sun’s surface with tremendous energy.
However, as the wind travels away from the Sun, its fine details become blurred, like the jets of water merging into a single stream.
The Parker Solar Probe has managed to reach close enough to the Sun to witness these hidden granular features, which are lost once the solar wind exits the corona, the Sun’s outer atmosphere.
In a recently published study in the journal Nature, a team of scientists led by Professor Stuart D. Bale from the University of California, Berkeley, and James Drake from the University of Maryland-College Park, shares their remarkable discoveries.
The Parker Solar Probe detected streams of high-energy particles that align with the supergranulation flows within coronal holes, revealing that these regions are the birthplace of the so-called “fast” solar wind.
Coronal holes are areas on the Sun’s surface where magnetic field lines emerge and expand outward without looping back.
During periods of solar inactivity, these holes are typically found at the poles and produce solar winds that do not impact Earth directly.
However, when the Sun becomes active every 11 years, these holes appear all over the Sun’s surface, generating bursts of solar wind directed towards Earth.
Understanding the origins of solar wind is crucial for predicting and preparing for solar storms. While these storms create mesmerizing auroras on Earth, they can also disrupt satellites and electrical grids, posing risks to our communication networks and daily lives.
Drake emphasizes the significance of comprehending the sun’s wind mechanism, stating, “Winds carry lots of information from the sun to Earth, so understanding the mechanism behind the sun’s wind is important for practical reasons on Earth.
That’s going to affect our ability to understand how the sun releases energy and drives geomagnetic storms, which are a threat to our communication networks.”
The researchers’ analysis suggests that the coronal holes resemble showerheads, with evenly spaced jets emanating from bright spots where magnetic field lines penetrate and exit the Sun’s surface.
They propose that when oppositely directed magnetic fields interact in these areas, known as funnels, they often break and reconnect, propelling charged particles outward.
Bale explains, “The photosphere, the Sun’s visible surface, is covered by convection cells similar to boiling water.
The larger-scale convection flow is called supergranulation. Where these supergranulation cells converge and descend, they drag the magnetic field into a downward funnel.
The magnetic field intensifies in these regions because it’s compressed, like a bundle of magnetic field lines going down a drain. The spatial separation of these funnels, or drains, is what we can now observe with the Parker Solar Probe data.”
Based on the detection of extremely high-energy particles by the Parker Solar Probe—particles traveling 10 to 100 times faster than the average solar wind—the scientists conclude that the fast solar wind is primarily formed through a process called magnetic reconnection.
This process takes place within the funnel structures in the coronal holes. The Parker Solar Probe, launched in 2018, was specifically designed to address the debate between two explanations for the origin of high-energy solar wind particles: magnetic reconnection or acceleration by plasma or Alfvén waves.
Bale asserts, “The significant conclusion is that magnetic reconnection within these funnel structures is the energy source for the fast solar wind. It does not come from everywhere within a coronal hole but is substructured within these supergranulation cells. It arises from these small bundles of magnetic energy associated with the convection flows. Our results provide strong evidence for the role of magnetic reconnection in this process.”
These funnel structures likely correspond to the bright jetlets observed within coronal holes from Earth, as recently reported by Nour Raouafi, a co-author of the study and the Parker Solar Probe project scientist at the Applied Physics Laboratory at Johns Hopkins University.
As the solar wind reaches Earth, approximately 93 million miles away from the Sun, it transforms into a turbulent flow of magnetic fields and charged particles that interact with Earth’s own magnetic field.
This interaction releases electrical energy into the upper atmosphere, generating captivating auroras near the poles.
However, the effects of intense solar storms can extend to our atmosphere, affecting various aspects of our modern technology. Predicting these solar storms and their impact on Earth is a crucial aspect of NASA’s Living With a Star program, which funded the Parker Solar Probe mission.
To capture a comprehensive view of the turbulent solar wind at its point of origin near the Sun’s surface, PSP had to approach within a distance of 25 to 30 solar radii, which is around 13 million miles.
Bale explains, “Once you get below that altitude, 25 or 30 solar radii or so, there’s a lot less evolution of the solar wind, and it’s more structured—you see more of the imprints of what was on the Sun.”
In 2021, PSP’s instruments recorded magnetic field switchbacks in the Alfvén waves associated with the regions where the solar wind is generated. By the time the probe reached a distance of approximately 5.2 million miles from the Sun’s surface, the data clearly showed that the probe was traversing jets of material rather than mere turbulence.
Bale, Drake, and their colleagues traced these jets back to the supergranulation cells in the Sun’s photosphere, where magnetic fields bunch up and funnel into the Sun.
The detection of extremely high-energy particles in these jets, ranging from tens to hundreds of kiloelectron volts (keV) compared to the few keV of most solar wind particles, supports the theory that magnetic reconnection accelerates the particles and generates Alfvén waves, providing an additional boost to the particles.
Bale explains their interpretation, stating, “Our conclusion is that these jets of reconnection outflow excite Alfvén waves as they propagate out. This observation is well-known in Earth’s magnetotail, where similar processes occur. I don’t understand how wave damping can produce these hot particles up to hundreds of keV, whereas it comes naturally out of the reconnection process. And we see it in our simulations too.”
Unfortunately, the Parker Solar Probe cannot get closer than approximately 4 million miles, or 8.8 solar radii, to the Sun’s surface without risking damage to its instruments.
However, Bale anticipates that the team will strengthen their conclusions with data from this altitude. It’s worth noting that the Sun is now entering a more chaotic phase known as solar maximum, which might complicate the scientists’ efforts to observe the processes they seek.
Bale concludes, “There was some concern at the beginning of the solar probe mission that we were launching it during the quietest, least eventful part of the solar cycle.
But I believe that without this timing, we would have never gained this understanding. It would have been too complex. I consider us fortunate to have launched the probe during the solar minimum.”