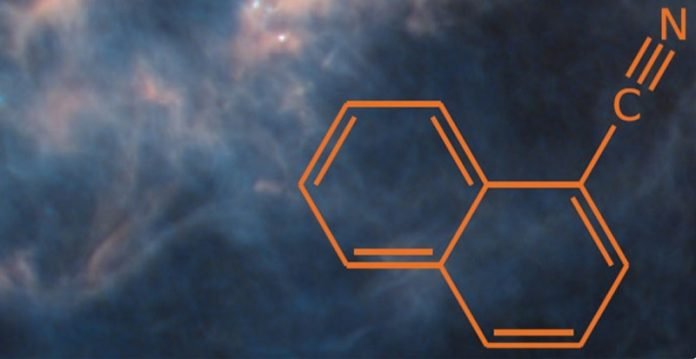
Whether peering through a backyard telescope or glancing at the majestic photographs taken by the James Webb telescope, the beauty of the cosmos continues to capture the imagination of children and adults alike.
One of the questions that have baffled astronomers and chemists for several decades is the structure and lifecycle of the molecules out there.
In 2021, after decades of searching, matching of high-resolution spectroscopic data to radioastronomy observations unequivocally identified two forms (isomers) of cyanonaphthalene – molecules that have the same backbone as the active ingredient in mothballs – in the Taurus Molecular Cloud-1 (TMC-1).
These PAH molecules and their larger siblings are surmised to constitute more than 15% of galactic carbon.
However, the cyanonaphthalene molecules were a million times more abundant than state-of-the-art astrochemical modeling could predict, revealing gaping chasms in our understanding of the chemistry in these extreme environments.
A recent study, published as part of an international collaboration between the Bull group UEA and researchers in the Physics Department at Stockholm University, provides an answer to the question:
How do small PAH molecules survive in space?
Using the DESIREE infrastructure in Sweden, which generates conditions that emulate those in outer space, the researchers found that a significant part of the modeling discrepancy was due to neglect of radiative stabilization by Recurrent Fluorescence (RF) – the emission of optical photons from thermally excited electronic states.
The study has been published in Nature Communications.
The team pioneered a new technique for determining absolute dissociation and RF stabilization rates by analyzing the kinetic energy release distributions of the fragments emitted when highly-excited cyanonaphthalene ions disintegrated.
Crucially, the authors found that the RF rate is boosted by more than two orders of magnitude by vibronic coupling due to the mixing of electronic and nuclear motions.
These ground-breaking results call for reconsideration of long-standing hypotheses regarding the resilience of small PAH molecules in space.
A follow-up article has been accepted for publication in Faraday Discussions.
This work helps lay a solid foundation for understanding how and why certain molecules exist in space while others do not.
Funded by a collaborative early career research grant, the team is currently analyzing and preparing results from other specialized facilities across Europe.