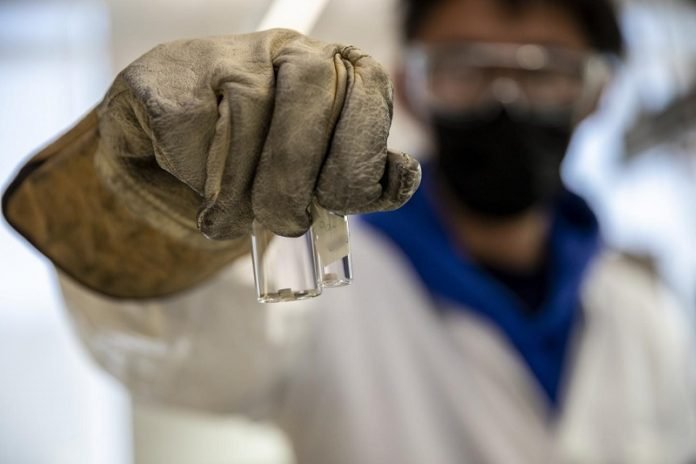
In our future electrified world, the demand for battery storage is projected to be enormous, reaching to upwards of 2 to 10 terawatt-hours (TWh) of annual battery production by 2030, from less than 0.5 TWh today.
However, concerns are growing as to whether key raw materials will be adequate to meet this future demand.
The lithium-ion battery – the dominant technology for the foreseeable future – has a component made of cobalt and nickel, and those two metals face severe supply constraints on the global market.
Now, after several years of research led by Lawrence Berkeley National Laboratory (Berkeley Lab), scientists have made significant progress in developing battery cathodes using a new class of materials that provide batteries with the same if not higher energy density than conventional lithium-ion batteries but can be made of inexpensive and abundant metals.
Known as DRX, which stands for disordered rocksalts with excess lithium, this novel family of materials was invented less than 10 years ago and allows cathodes to be made without nickel or cobalt.
“The classic lithium-ion battery has served us well, but as we consider future demands for energy storage, its reliance on certain critical minerals exposes us not only to supply-chain risks, but also environmental and social issues,” said Ravi Prasher, Berkeley Lab’s Associate Lab Director for Energy Technologies.
“With DRX materials, this offers lithium batteries the potential to be the foundation for sustainable battery technologies for the future.”
The cathode is one of the two electrodes in a battery and accounts for more than one-third of the cost of a battery. Currently the cathode in lithium-ion batteries uses a class of materials known as NMC, with nickel, manganese, and cobalt as the key ingredients.
“I’ve done cathode research for over 20 years, looking for new materials, and DRX is the best new material I’ve ever seen by far,” said Berkeley Lab battery scientist Gerbrand Ceder, who is co-leading the research.
“With the current NMC class, which is restricted to just nickel, cobalt, and an inactive component made of manganese, the classic lithium-ion battery is at the end of its performance curve unless you transfer to new cathode materials, and that’s what the DRX program offers.
DRX materials have enormous compositional flexibility – and this is very powerful because not only can you use all kinds of abundant metals in a DRX cathode, but you can also use any type of metal to fix any problem that might come up during the early stages of designing new batteries. That’s why we’re so excited.”
Cobalt and nickel supply-chain risks
The U.S. Department of Energy (DOE) has made it a priority to find ways to reduce or eliminate the use of cobalt in batteries. “The battery industry is facing an enormous resource crunch,” said Ceder.
“Even at 2 TWh, the lower range of global demand projections, that would consume almost all of today’s nickel production, and with cobalt we’re not even close. Cobalt production today is only about 150 kilotons, and 2 TWh of battery power would require 2,000 kilotons of nickel and cobalt in some combination.”
What’s more, over two-thirds of the world’s nickel production is currently used to make stainless steel. And more than half of the world’s production of cobalt comes from the Democratic Republic of Congo, with Russia, Australia, the Philippines, and Cuba rounding out the top five producers of cobalt.
In contrast, DRX cathodes can use just about any metal in place of nickel and cobalt. Scientists at Berkeley Lab have focused on using manganese and titanium, which are both more abundant and lower cost than nickel and cobalt.
“Manganese oxide and titanium oxide cost less than $1 per kilogram whereas cobalt costs about $45 per kilogram and nickel about $18,” said Ceder. “With DRX you have the potential to make very inexpensive energy storage. At that point lithium-ion becomes unbeatable and can be used everywhere – for vehicles, the grid – and we can truly make energy storage abundant and inexpensive.”
Ordered vs. disordered
Ceder and his team developed DRX materials in 2014. In batteries, the number and speed of lithium ions able to travel into the cathode translates into how much energy and power the battery has.
In conventional cathodes, lithium ions travel through the cathode material along well-defined pathways and arrange themselves between the transition metal atoms (usually cobalt and nickel) in neat, orderly layers.
What Ceder’s group discovered was that a cathode with a disordered atomic structure could hold more lithium – which means more energy – while allowing for a wider range of elements to serve as the transition metal. They also learned that within that chaos, lithium ions can easily hop around.
In 2018, the Vehicle Technologies Office in DOE’s Office of Energy Efficiency and Renewable Energy provided funding for Berkeley Lab to take a “deep dive” into DRX materials.
In collaboration with scientists at Oak Ridge National Laboratory, Pacific Northwest National Laboratory, and UC Santa Barbara, Berkeley Lab teams led by Ceder and Guoying Chen have made tremendous progress in optimizing DRX cathodes in lithium-ion batteries.
For example, the charge rate – or how fast the battery can charge – of these materials was initially very low, and its stability was also poor. The research team has found ways to address both of these issues through modeling and experimentation.
Studies on using fluorination to improve stability have been published in Advanced Functional Materials and Advanced Energy Materials; research on how to enable a high charging rate was recently published in Nature Energy.
Since DRX can be made with many different elements, the researchers have also been working on which element would be best to use, hitting the sweet spot of being abundant, inexpensive, and providing good performance. “DRX has now been synthesized with almost the whole periodic table,” Ceder said.
“This is science at its best – fundamental discoveries that will serve as the bedrock of systems in future homes, vehicles, and grids,” said Noel Bakhtian, director of Berkeley Lab’s Energy Storage Center.
“What has made Berkeley Lab so successful in battery innovation for decades now is our combination of breadth and depth of expertise – from fundamental discovery to characterization, synthesis, and manufacturing, as well as energy markets and policy research.
Collaboration is key – we partner with industry and beyond to solve real-world problems, which in turn helps galvanize the world-leading science we do at the Lab.”
Fast progress
New battery materials have traditionally taken 15 to 20 years to commercialize; Ceder believes progress on DRX materials can be accelerated with a larger team.
“We’ve made great progress in the last three years with the deep dive,” Ceder said. “We’ve come to the conclusion that we’re ready for a bigger team, so we can involve people with a more diverse set of skills to really refine this.”
An expanded research team could move quickly to address the remaining issues, including improving the cycle life (or the number of times the battery can be recharged and discharged over its lifetime) and optimizing the electrolyte, the chemical medium that allows the flow of electrical charge between the cathode and anode.
Since being developed in Ceder’s lab, groups in Europe and Japan have also launched large DRX research programs.
“Advances in battery technologies and energy storage will require continued breakthroughs in the fundamental science of materials,” said Jeff Neaton, Berkeley Lab’s Associate Lab Director for Energy Sciences.
“Berkeley Lab’s expertise, unique facilities, and capabilities in advanced imaging, computation, and synthesis allow us to study materials at the scale of atoms and electrons. We are well poised to accelerate the development of promising materials like DRX for clean energy.”