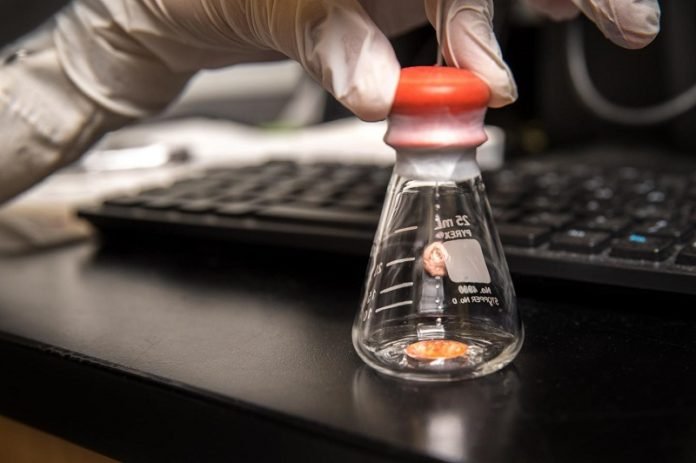
Scientists have discovered the root cause of why lithium metal batteries fail.
Bits of lithium metal deposits break off from the surface of the anode during discharging and are trapped as “dead” or inactive lithium that the battery can no longer access.
The discovery, published in Nature, challenges the conventional belief that lithium metal batteries fail because of the growth of a layer, called the solid electrolyte interphase (SEI), between the lithium anode and the electrolyte.
The researchers made their discovery by developing a technique to measure the amounts of inactive lithium species on the anode–a first in the field of battery research–and studying their micro- and nanostructures.
The findings could pave the way for bringing rechargeable lithium metal batteries from the lab to the market.
“By figuring out the major underlying cause of lithium metal battery failure, we can rationally come up with new strategies to solve the problem,” said first author Chengcheng Fang, a materials science and engineering Ph.D. student at UC San Diego.
“Our ultimate goal is to enable a commercially viable lithium metal battery.”
Lithium metal batteries, which have anodes made of lithium metal, are an essential part of the next generation of battery technologies.
They promise twice the energy density of today’s lithium-ion batteries (which usually have anodes made of graphite), so they could last longer and weigh less. This could potentially double the range of electric vehicles.
But a major issue with lithium metal batteries is low Coulombic efficiency, meaning they undergo a limited number of cycles before they stop working.
That’s because as the battery cycles, its stores of active lithium and electrolyte get depleted.
Battery researchers have long suspected that this is due to the growth of the solid electrolyte interphase (SEI) layer between the anode and the electrolyte.
But although researchers have developed various ways to control and stabilize the SEI layer, they still have not fully resolved the problems with lithium metal batteries, explained senior author Y. Shirley Meng, a nanoengineering professor at UC San Diego.
“The cells still fail because a lot of inactive lithium is forming in these batteries. So there is another important aspect that is being overlooked,” Meng said.
The culprits, Meng, Fang and colleagues found, are lithium metal deposits that break off of the anode when the battery is discharging and then get trapped in the SEI layer.
There, they lose their electrical connection to the anode, becoming inactive lithium that can no longer be cycled through the battery. This trapped lithium is largely responsible for lowering the Coulombic efficiency of the cell.
Measuring the ingredients of inactive lithium
The researchers identified the culprit by creating a method to measure how much unreacted lithium metal gets trapped as inactive lithium.
Water is added to a sealed flask containing a sample of inactive lithium that formed on a cycled half-cell.
Any bits of unreacted lithium metal chemically react with water to produce hydrogen gas. By measuring how much gas is produced, researchers can calculate the amount of trapped lithium metal.
Inactive lithium is also made up of another component: lithium ions, which are the building blocks of the SEI layer. Their amount can also be calculated simply by subtracting the amount of unreacted lithium metal from the total amount of inactive lithium.
In tests on lithium metal half-cells, researchers found that unreacted lithium metal is the main ingredient of inactive lithium. As more of it forms, the lower the Coulombic efficiency sinks. Meanwhile, the amount of lithium ions from the SEI layer consistently stays low. These results were observed in eight different electrolytes.
“This is an important finding because it shows that the primary failure product of lithium metal batteries is unreacted metallic lithium instead of the SEI,” Fang said.
“This is a reliable method to quantify the two components of inactive lithium with ultra-high accuracy, which no other characterization tool has been capable of doing.”
“The aggressive chemical nature of lithium metal has made this task very challenging. Parasitic reactions of many different types occur simultaneously on lithium metal, making it almost impossible to differentiate these different types of inactive lithium,” said Kang Xu, whose team at the U.S. Army Combat Capabilities Development Command Army Research Laboratory provided one of the advanced electrolyte formulations to test the method.
“The advanced methodology established in this work provides a very powerful tool to do this in a precise and reliable way.”
The researchers hope their method could become the new standard for evaluating efficiency in lithium metal batteries.
“One of the problems battery researchers face is that testing conditions are very different across labs, so it’s hard to compare data. It’s like comparing apples to oranges.
Our method can enable researchers to determine how much inactive lithium forms after electrochemical testing, regardless of what type of electrolyte or cell format they use,” Meng said.
A closer look at inactive lithium
By studying the micro- and nanostructures of lithium deposits in different electrolytes, the researchers answer another important question: why some electrolytes improve Coulombic efficiency while others do not.
The answer has to do with how lithium deposits on the anode when the cell is charging. Some electrolytes cause lithium to form micro- and nanostructures that boost cell performance.
For example, in an electrolyte specially designed by Meng’s collaborators at General Motors, lithium deposits as dense, column-shaped chunks.
This type of structure causes less unreacted lithium metal to get trapped in the SEI layer as inactive lithium during discharge. The result is a Coulombic efficiency of 96 percent for the first cycle.
“This excellent performance is attributed to the columnar microstructure formed on the surface of the current collector with minimum tortuosity, which significantly enhances the structural connection,” said Mei Cai, whose team at General Motors developed the advanced electrolyte that enabled lithium to deposit with the “ideal” microstructure.
In contrast, when a commercial carbonate electrolyte is used, lithium deposits with a twisty, whisker-like morphology.
This structure causes more lithium metal to get trapped in the SEI during the stripping process. Coulombic efficiency lowers to 85 percent.
Moving forward, the team proposes strategies to control the depositing and stripping of lithium metal. These include applying pressure on the electrode stacks; creating SEI layers that are uniform and mechanically elastic; and using 3D current collectors.
“Control of the micro- and nanostructure is key,” Meng said. “We hope our insights will stimulate new research directions to bring rechargeable lithium metal batteries to the next level.”
Written by Liezel Labios.